Introduction
Many advanced ceramics display electrical properties superior to or unattainable in their metallic and polymeric counterparts; these materials are known as electroceramics. One group of electroceramics — referred to alternatively as "fast ion conductors", "rapid ion conductors" or "superionic conductors" — are able to support a high flux of ions (charged atoms) in the solid state. Ceramics that rapidly conduct oxide ions (O2−) can be used as solid electrolytes in a number of industrial applications including oxygen gas sensors, oxygen pumps, exhaust catalysts and solid oxide fuel cells (SOFCs). Solid-state scientists and materials engineers are working feverishly to make these efficient, environmentally-friendly devices a reality for tomorrow's industries and consumers.
Information about advanced ceramics in Australia and the UK.
What are Fuel Cells?
A fuel cell is an energy conversion device that generates electricity and heat by electrochemically combining a gaseous fuel and an oxidizing gas via an ion-conducting electrolyte. The chief characteristic of a fuel cell is its ability to convert chemical energy directly into electrical energy without the need for combustion, giving much higher conversion efficiencies than conventional thermo-mechanical methods (eg steam turbines and internal combustion engines). Consequently fuel cells have much lower carbon dioxide emissions than fossil fuel-based technologies for the same power output. They also produce negligible amounts of SOx and NOx, the main constituents of acid rain and photochemical smog.
The concept of a fuel cell was first expounded by Sir William Grove in 1839, but it has only been in the last few decades that research into fuel cells has (literally) taken off. Several types of fuel cells are now being developed around the world, the chief difference between them being the material used for the electrolyte (and hence also their operating temperature). Small, alkaline fuel cells (utilizing a liquid electrolyte) were developed by NASA to power the Apollo and other space missions in the 1960s, and improved versions are still used on the Space Shuttle today. SOFCs, in contrast, are constructed entirely from solid-state materials; they utilize a fast oxide-ion−conducting ceramic as the electrolyte, and operate in the temperature range 900–1000oC. SOFCs provide the following advantages compared with other fuel cell types:




SOFC Design and Operation
A single SOFC unit consists of two electrodes (an anode and cathode) separated by the electrolyte (Figure 1). Fuel (usually hydrogen, H2, or methane, CH4) arrives at the anode, where it reacts with oxide ions from the electrolyte, thereby releasing electrons (e−) to the external circuit. On the other side of the fuel cell, oxidant (usually O2 or air) is fed to the cathode, where it supplies the oxide ions (O2−) for the electrolyte by accepting electrons from the external circuit. The electrolyte conducts these ions between the electrodes, maintaining overall electrical charge balance. The flow of electrons in the external circuit provides useful power.
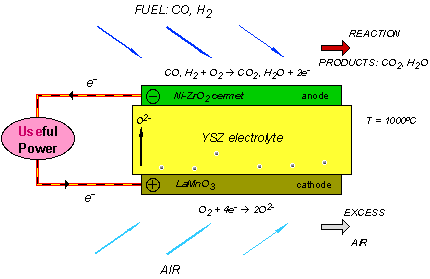
Figure 1: Concept diagram for a solid oxide fuel cell
Today's technology employs several ceramic materials as the active SOFC components. The anode is typically formed from an electronically conducting nickel/yttria-stabilised zirconia (Ni/YSZ) cermet (ie, a ceramic/metal composite). The cathode is based on a mixed (ie, both electronic and ionic) conducting perovskite, lanthanum manganate (LaMnO3). Yttria-stabilised zirconia (YSZ) is used for the oxide-ion−conducting electrolyte. To generate a reasonable voltage, fuel cells are not operated as single units but as an array of units or "stack", with a doped lanthanum chromite (eg, La0.8Ca0.2CrO3) interconnect joining the anodes and cathodes of adjacent units. Although several stack designs are being considered around the world, the most common configuration is the planar (or "flat-plate") SOFC illustrated in Figure 2.
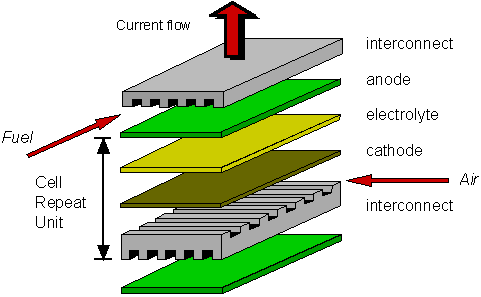
Figure 2: Planar design for a solid oxide fuel cell (exploded view)
Future Development of SOFCs
For the YSZ electrolyte to provide sufficient ionic conductivity, it must be heated to high temperature (900–1000oC). This means that expensive superalloys must be used to house the fuel cell, increasing its cost substantially. These costs could be reduced if the operating temperature were lowered to between 600 and 800oC, allowing the use of cheaper structural components such as stainless steel. A lower operating temperature would also ensure a greater overall system efficiency and a reduction in the thermal stresses in the active ceramic structures, leading to a longer service life for the system.
To lower the operating temperature, either the conductivity of YSZ must be improved, or alternative electrolytic materials must be developed that can replace YSZ. One way of improving the conductivity is to make the electrolyte thinner; the shorter the distance the oxide ions have to travel, the less overall resistance they encounter. Nowadays state-of-the-art thin film technology is used to produce electrolytes between 10 and 100 microns (0.01 to 0.1 mm) thick. The major challenge is to ensure that the electrolyte remains fully dense so that the system isn't short-circuited by gases passing from one side of the cell to the other.
A concerted effort is also being made to develop new electrolyte materials. Ceramics that are currently being investigated include Gd-doped CeO2, Ba2In2O5 and (Sr,Mg)-doped LaGaO3 (LSGM). However, these "novel" materials all face serious drawbacks compared with YSZ, and it is most likely that the first commercial SOFC units will use zirconia-based ceramics as the electrolyte.
Links
For more information on fuel cells, check out these sites:



















